OVERVIEW
Three-Dimensional Printing Technology
(Editor's Note: In this regular column, JCO provides an overview of a clinical topic of interest to orthodontists. Contributions and suggestions for future subjects are welcome.)
Recent advances in intraoral digital scanning technology have given orthodontists the ability to eliminate unpleasant impressions while providing patients with more accurate appliances and reduced treatment times.1 The next paradigm shift in orthodontics will come with the development of three-dimensional printers, working in conjunction with intraoral scanners.
Also known as additive manufacturing, 3D printing is a technology whereby sequential layers of material are deposited on top of one another to eventually form an object.2 It is the opposite of subtractive manufacturing, in which a block of material is carved away to form the object (as with milling units such as the chairside economical restoration of esthetic ceramics, or CEREC). Three-dimensional printing is becoming commonplace in medicine for custom fabrication of prostheses to replace missing tissues,3 and even to provide scaffolding for tissue engineering.4 Although subtractive manufacturing has long been the technology of choice in dentistry, it is only a matter of time before 3D printing becomes the standard.5 Orthodontists are already familiar with several products that use 3D printers, including Invisalign* and ClearCorrect**.6
Similar articles from the archive:
The concept of 3D printing was first developed by Chuck Hull in 1984, when he was using ultraviolet light to cure tabletop coatings. In 1986, Hull established the 3D Systems*** company to market the first machine for rapid prototyping, which he called stereolithography (SLA).7 In 1988, Scott Crump developed fused deposition modeling (FDM), which was commercialized by Stratasys† in 1990. Objet Geometries†, the developer of PolyJet† photopolymer (PPP) printing, was founded in 1998. Dozens of 3D printers employing variations of SLA, FDM, and PolyJet technologies are now available from many different companies.
The typical two-dimensional printer used for printing on paper works along the right-to-left x-axis and the top-to-bottom y-axis. In 3D printing, the up-and-down z-axis is added. An intraoral scanner is used to create a digital file of the object in standard tessellation language (STL), the global format for 3D printing files. Computer-aided design and manufacturing (CAD/CAM) software is used to further process the file and prepare it for printing. The software then breaks down the object into small layers of 16-300 microns each, known as "build layers". The time required to produce 3D models depends on the number of layers being printed--the vertical height of the model--rather than the number of models being printed. For example, one 5cm-tall model may take six hours to print, while 10 1cm-tall models will take about one hour.
While technologies differ, every 3D printer requires a computer workstation to set up the print job (some integrated with the printer itself); a build tray for fabricating the model; and a print medium, which is either unwound from a spool, dispensed from a sealed container, or poured from a container into a vat. The print medium may be any of a number of materials, including plastic, metal, clay, sand, and even human cells, each of which is best suited to a specific type of printer. This article will highlight the most common types of 3D printing technology for orthodontic use.
Stereolithography
Although 3D Systems is still the largest producer of SLA printers, many other models are currently available. The build tray of an SLA printer is immersed in a liquid resin that is curable by a concentrated ultraviolet laser light (Fig. 1).8
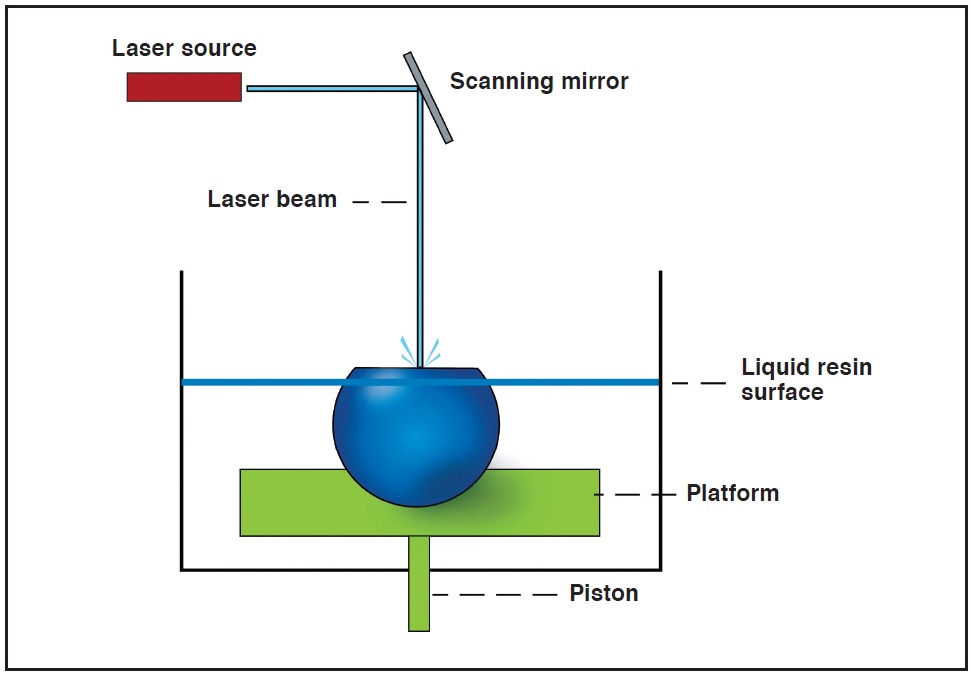
Fig. 1 Stereolithography (SLA) printer.
The laser draws a cross-section of the object to form each layer. After the layer is cured, the tray descends by a distance equal to the layer thickness, allowing uncured resin to cover the previous layer. This process is repeated hundreds of times as the printed object takes shape. SLA printers are generally slower than others because the laser can cure only a small area at a time.
Fused Deposition Modeling
The patents originally held by Stratasys have expired--resulting in dozens of FDM brands for the consumer market--but Stratasys still offers a line of FDM printers. Instead of curing a liquid resin with projected light, an FDM printer extrudes a resin that has been heated just beyond its melting point, depositing it layer by layer (Fig. 2).
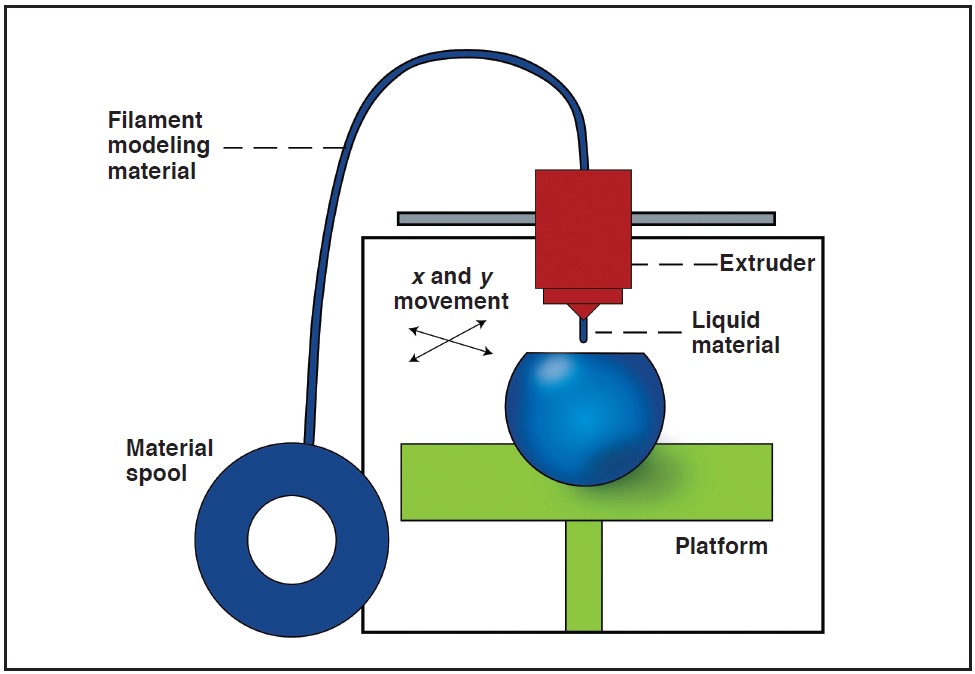
Fig. 2 Fused deposition modeling (FDM) printer.
The heated material hardens immediately after being extruded, thus minimizing inaccuracies. Of the available materials, the most common are polylactic acid and acrylonitrile butadiene styrene (ABS). These often come on spools that can easily be changed as necessary.
Digital Light Processing
EnvisionTEC‡ is the largest of many producers of Digital Light Processing (DLP)†† printers. DLP is identical to SLA except for the light source: a projector is used to cure an entire layer at a time (Fig. 3), in contrast to the SLA laser, which must draw the entire layer to cure it.
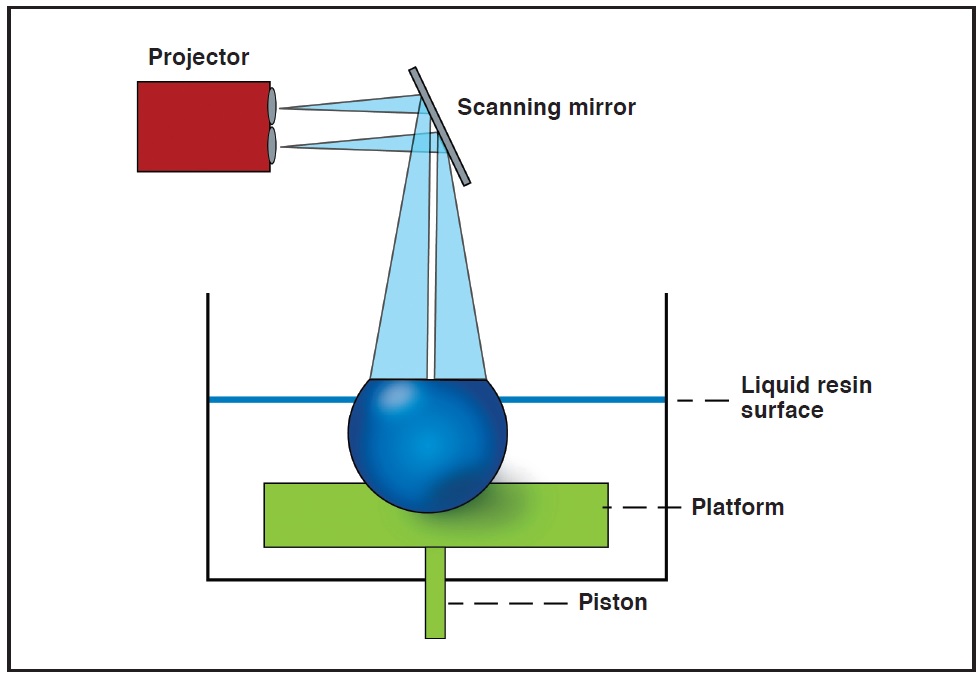
Fig. 3 Digital Light Processing (DLP) printer.
Similar to the difference between stamping and drawing an object, this results in significantly faster print times. The heart of the DLP projector is a chip developed by Texas Instruments. Known as a digital micro-mirror device, the chip contains hundreds of thousands of tiny mirrors that are able to move in two directions, on and off, thousands of times per second. Because a DLP printer builds a model in voxels rather than layers, there are no visible steps, making the finish quality the best of all 3D printing technologies. Many media are available for DLP printers, from ABS plastic to materials designed for burn-out casting.
PolyJet Photopolymerization
Stratasys and 3D Systems are currently the only manufacturers of PPP printers, which employ the same basic technology as the standard inkjet office printer, but in three dimensions. A liquid resin is jetted out of hundreds of nozzles and immediately cured with ultraviolet light (Fig. 4).
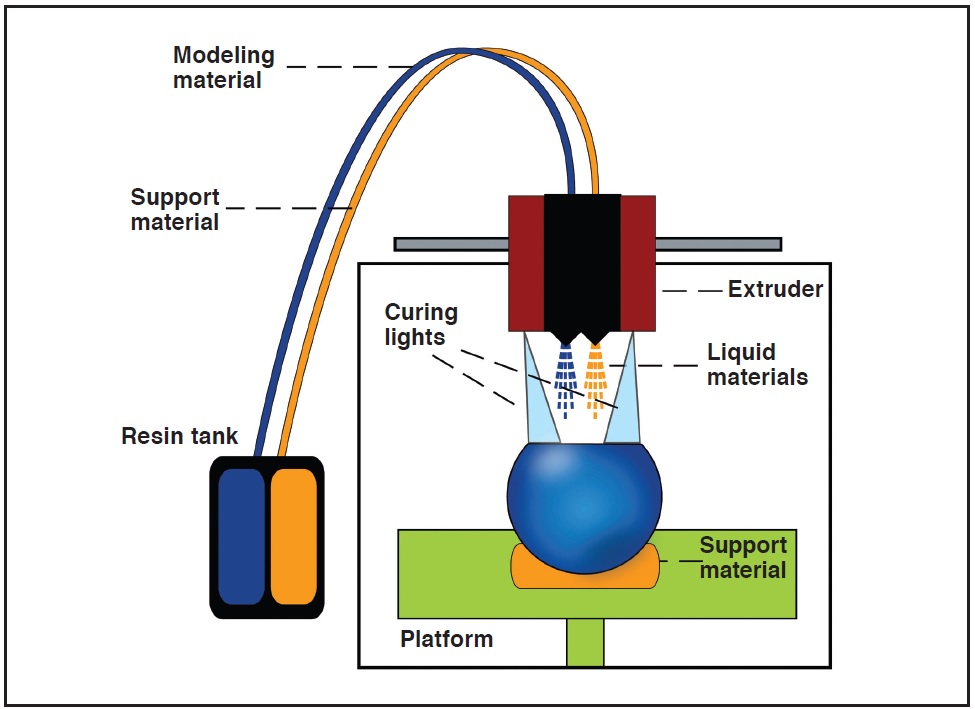
Fig. 4 PolyJet photopolymer (PPP) printer.
The build platform moves vertically to accommodate subsequent layers. Although this mechanism does leave stratification lines on the model, the build layers can be as thin as 16 microns, which means the surface quality of PPP models is often excellent. Higher-end PPP printers have the ability to print multiple materials on a single model. A wide variety of media are available, ranging from rubber- like compounds to materials designed to operate at high temperatures.
There is typically more waste with PPP printers than with other technologies, because a wiping blade is used before curing each layer to remove excess material and ensure dimensional accuracy, and because the print mechanism must occasionally be purged to ensure the print heads remain unclogged (even so, the print heads must be periodically replaced). Changing resins requires the lines to be purged of all material, resulting in the waste of unused resin. This material, which is not reusable or recyclable, is deposited in a waste tank that must be emptied regularly. While the volume of waste at any one time is minimal, it can add up during constant use.
Support Structures
Every 3D printer requires a support structure or support resin (Fig. 5), not only to prevent deflection due to gravity or movement by the printing mechanism, but also to enable the printing of complex objects with overhangs and undercuts.
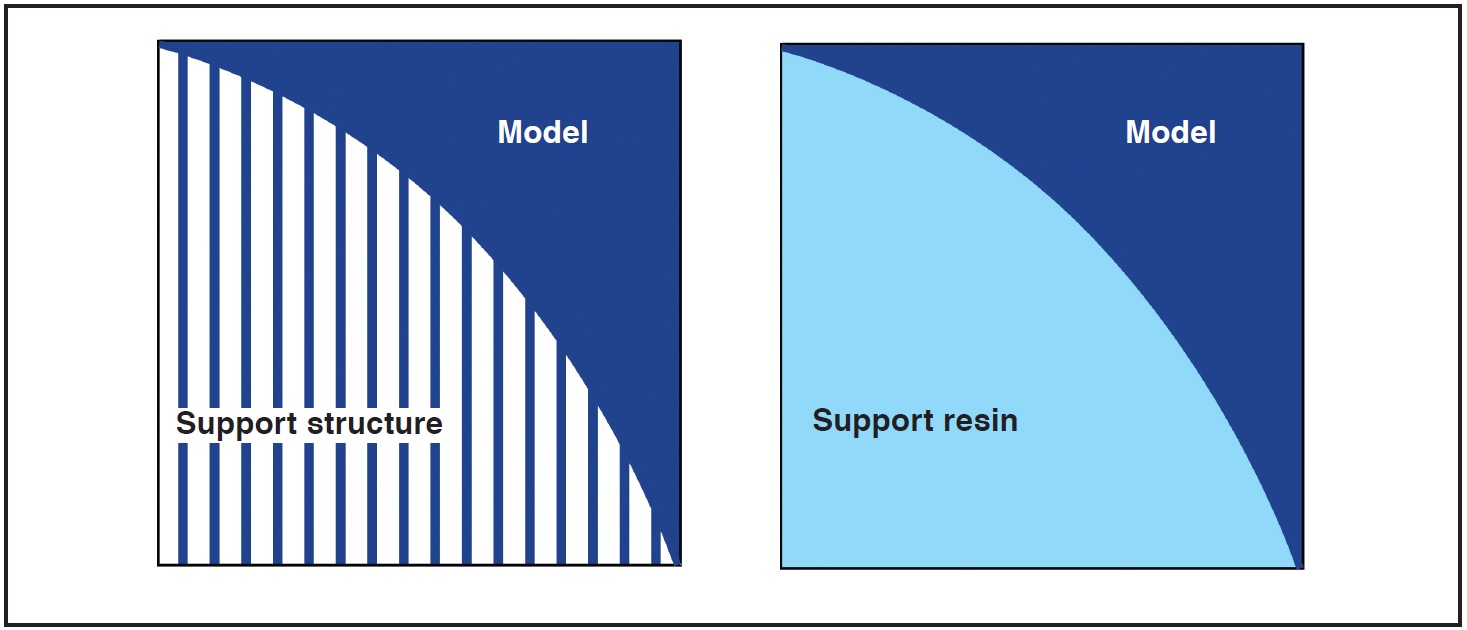
Fig. 5 Graphic comparison of support structure to support resin.
The printer software automatically adds these supports during model processing. SLA and DLP printers use perforated support structures, made from the model resin, that are easily removed after printing; PPP printers use a gel-like support resin that is removed with pressurized water.
Printers Used in Orthodontics
Objet30 OrthoDesk
The Objet30 OrthoDesk† (Table 1) is a new generation of the Stratasys Objet30 printer, which was developed as a smaller, more affordable PolyJet printer for small-business and home use. With a minimum layer thickness of 28 microns, the Objet30 can produce orthodontic models with excellent surface quality. Three materials are available: VeroDentPlus† (MED690), a peach-colored, acrylic-based polymer used for most appliances; MED610, a clear, biocompatible material commonly used for surgical guides and medically approved for temporary intraoral applications of as long as 24 hours; and VeroGlaze† (MED620), an acrylic used for veneer models or wax-ups in color A2 that can also be used in the mouth for 24 hours.
The build area for the OrthoDesk is 300mm x 200mm x 100mm, which allows as many as 12 horseshoe-shaped models to be printed in a single job (Fig. 6).
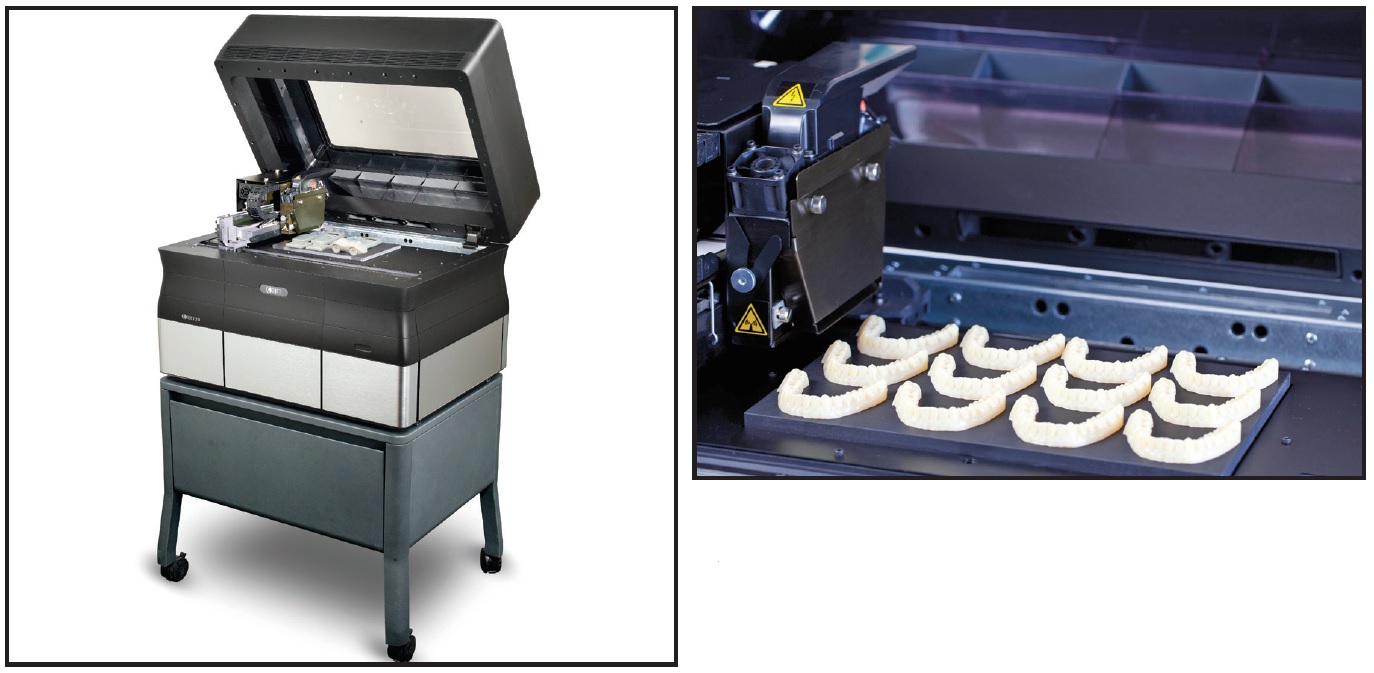
Fig. 6 Objet30 OrthoDesk printer and build area with printed models.
The OrthoDesk can print at 9mm (vertical height) per hour; an average single-arch model (without palatal coverage) costs $3-4. The support resin generally requires only a WaterJet washing station (supplied with the printer) to post-process the models. Alternatively, after a coarse removal of support material, the models can be soaked briefly in a mild "lye" solution and rinsed again with the WaterJet. The Objet30 OrthoDesk costs $44,295, including the printer and stand, model and support resin, WaterJet station, and installation and training.
Ultra 3SP Ortho
The Ultra 3SP Ortho‡ printer (Table 1) was designed specifically for dental use. Its proprietary 3SP (Scan, Spin, Selectively Photocure) technology, a variant of DLP introduced by EnvisionTEC in 2013, utilizes an orthogonal mirror that spins at 20,000rpm. A laser is reflected off the mirror and through a series of optics to focus the light on the uncured resin while moving across the build area. Compared to a conventional DLP printer with a static projector, this technology provides significantly faster print times: a maximum rate of 10mm per hour. Like other DLP printers, the Ultra 3SP builds models by voxels rather than layers, resulting in a superior surface finish. The build tray measures 266mm × 178mm × 76mm (Fig. 7).
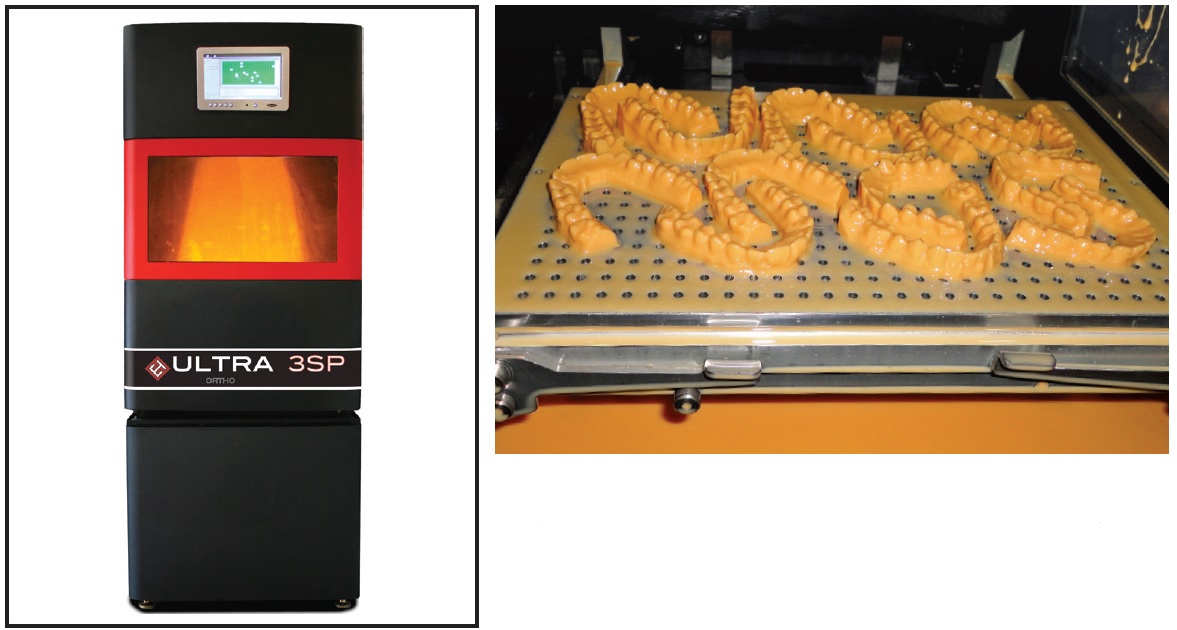
Fig. 7 Ultra 3SP Ortho printer and build area with printed models.
A common critique of DLP-based printers is that as the build envelope increases in size, the pixels become larger and accuracy declines. EnvisionTEC has developed two technologies--pixel shifting and gray scaling--to mitigate this projection effect. No support resin is required, but support structures can be added by the software. An alcohol solution must be used to clean the model, and an optional post-printing light-curing unit can be used for finishing.
While EnvisionTEC sells a variety of printing materials, the Ultra 3SP Ortho is limited to E-Denstone‡, the most popular medium for orthodontic use; ABS 3SP White‡, an ABS-like plastic; and E-Glass‡, a new, clear medium that can be used for a variety of applications. The biocompatible E-Dent‡ material is not available for the Ultra 3SP Ortho. The unit's base price is $38,900, but a full system costs about $47,950, including shipping, setup, start-up material, and two days of training.
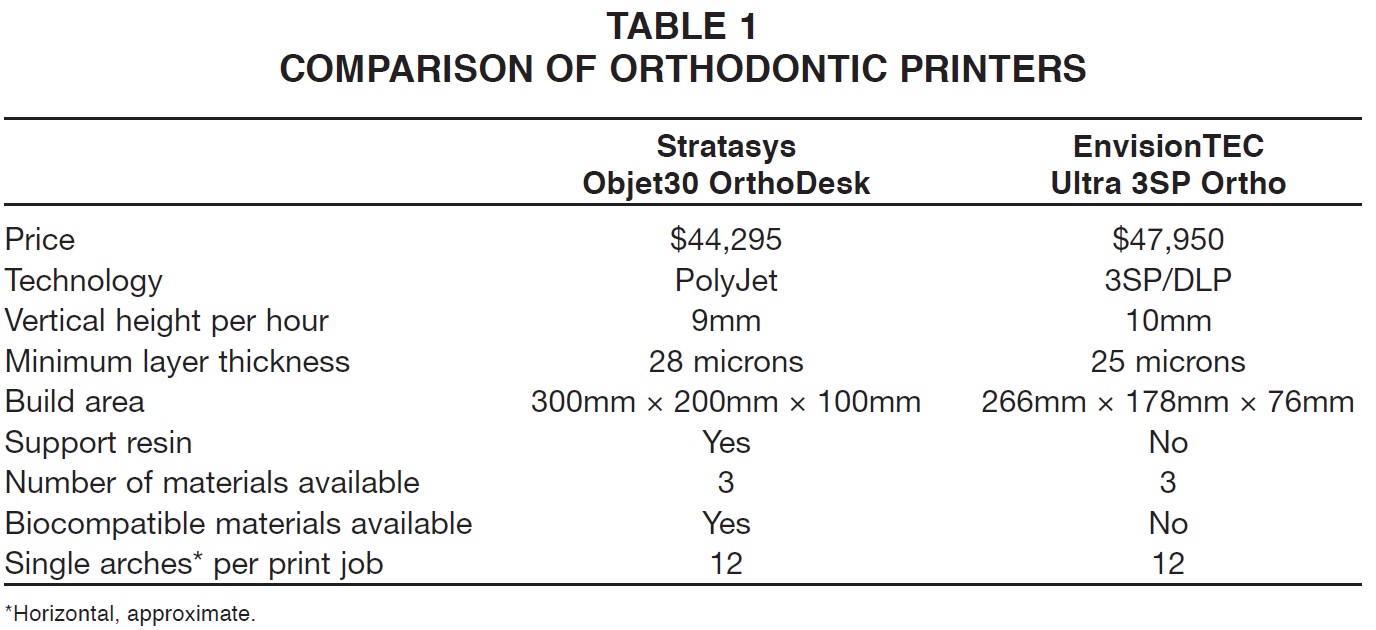
MakerBot and Other FDM Printers
FDM models are generally the least expensive of the 3D printers; the MakerBot Replicator 2‡‡, one of the most popular consumer brands, sells for $2,499. The materials are significantly less expensive as well: a 1kg spool of ABS Maker- Bot filament is $48, less than one-third the cost of materials for the Stratasys or EnvisionTEC printers. FDM printers are widely available, are easy to use and maintain, and have a smaller footprint than that of other printers. Due to the physical limitations of the extrusion nozzle, however, the layer size is larger, resulting in a less-than-ideal surface finish. For example, the Replicator 2 has a minimum layer size of 100 microns. While it is possible to fabricate retainers and aligners with these models, the visible stair-stepping makes appliances less esthetic and thus, perhaps, unacceptable to patients.
Because FDM printers such as the Replicator 2 were designed for hobbyists and not for the rigorous usage of an orthodontic office, reliability could also be an issue. Low-cost printers are often made with inexpensive parts that have the potential to break more easily. Even more problematic is the potential for slow degradation of parts over time, resulting in inaccuracies that are too small to detect visually but large enough to cause problems with appliance fit.
Materials Used in Orthodontics
The physical properties of the most common printer materials differ among manufacturers, further complicating the decision regarding which printer to buy. The American Society for Testing and Materials (ASTM) has developed international standards for testing these materials' physical properties--including tensile strength, tensile elongation, flexural strength, flexural modulus, and heat-deflection temperature--under identical conditions (Table 2).
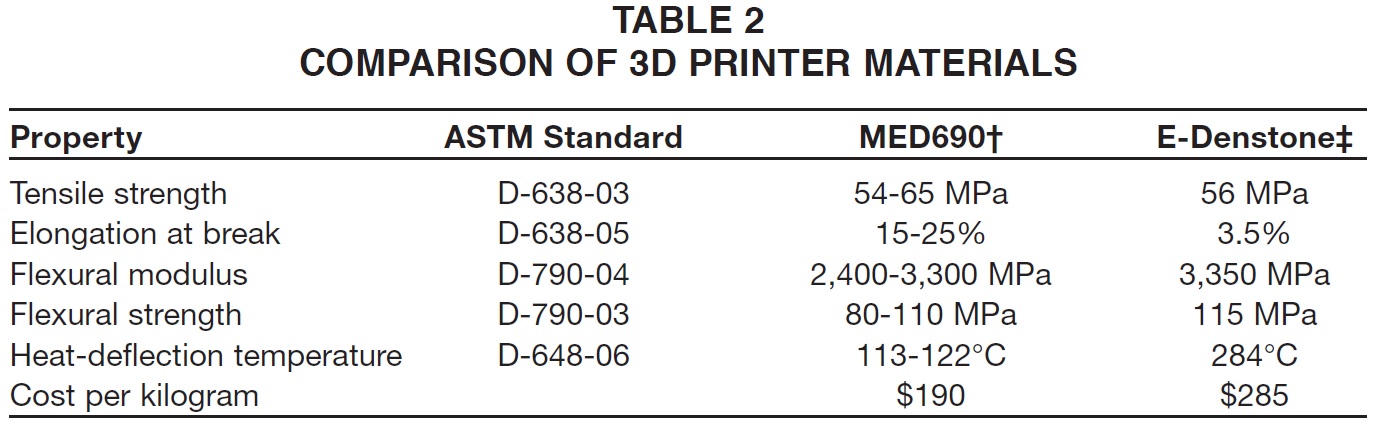
Although a material's response to tension is important, its response to flexural forces is even more significant in orthodontic applications, because a model will flex when the thermoformed appliance is removed. MED690 has a significantly higher elongation than E-Denstone 3SP at the break point, which means E-Denstone is more likely to fracture when a thermoformed appliance is removed.
It must be noted that the materials used in DLP/SLA printers are open to the environment, whereas the materials in PPP printers are fully enclosed. Before curing, these materials are slightly toxic. DLP/SLA materials come in containers for pouring into the printers' resin tanks; the manufacturers recommend wearing protective gloves and a mask whenever handling these resins. The inexpensive resins sold with consumer models contain higher levels of monomer that may not be completely cured upon printing, raising the question (untested by research) whether this leftover monomer could be transferred to an appliance and potentially become cytotoxic.
3D-Printed Models
Going from intraoral scan to physical model is not as simple as pressing "print" on your computer. In most cases, the digital model will have tiny holes that need to be stitched, closed, and repaired prior to printing. The software supplied with the printer is usually inadequate to prepare digital models, which require the aid of thirdparty CAD/CAM software such as NetFabb or Geomagic. This kind of program can be used to extend the model base, remove excess material, imprint a patient identifier, and extract only what the operator desires to print. By making the interior of the printed model hollow, for instance, the program conserves expensive resin. Prices of these third-party CAD/CAM programs range from $1,000-20,000, however, adding to the overall cost of 3D printing.
Digital models offer a unique opportunity to improve treatment efficiency. Using common CAD/ CAM tools, it is possible to digitally remove brackets prior to actual fixed-appliance removal. The operator must be careful not to create a divot in the model, since this will compromise the fit of the retainer. Once the brackets have been digitally removed, minor tooth movements can be prescribed to idealize alignment, and 3D models can be printed for retainer fabrication (Fig. 8). The main benefit of this protocol is that the removal appointment becomes substantially shorter when impressions do not need to be taken for in-office retainer fabrication. Moreover, since printed models are seldom damaged in making retainers, replacements can easily be fabricated as needed, as long as the patient has been diligent with retainer wear.
At this time, the most common orthodontic application of 3D printers is for the fabrication of clear retainers and aligner trays. A CAD/CAM program such as Orchestrate 3D§§§ is used to create sequential virtual setups, which are sent to the printer for model fabrication (Fig. 9).
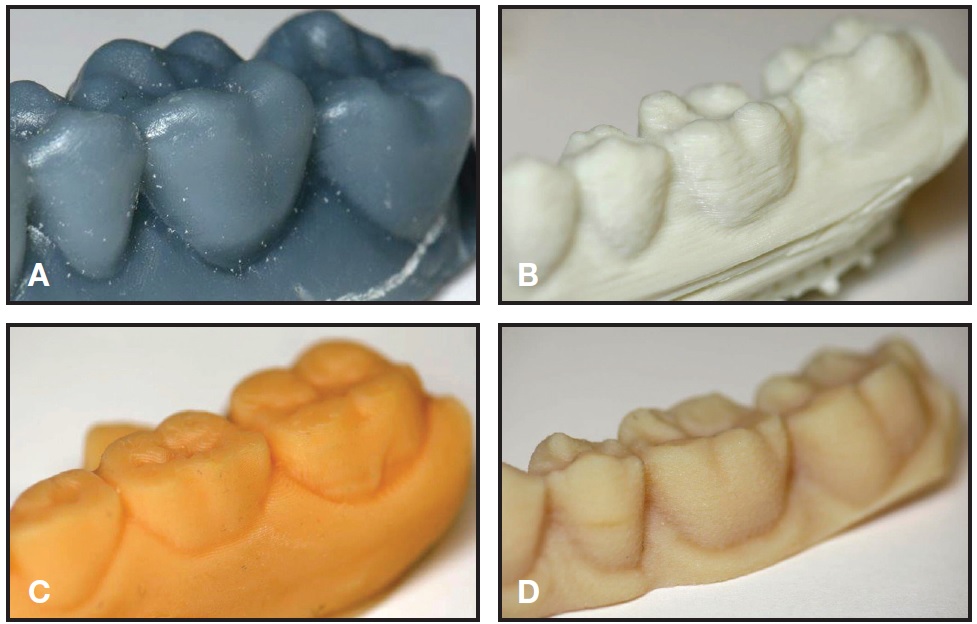
Fig. 8 Models printed using SLA (A), FDM (B), DLP (C), and PPP (D) systems.
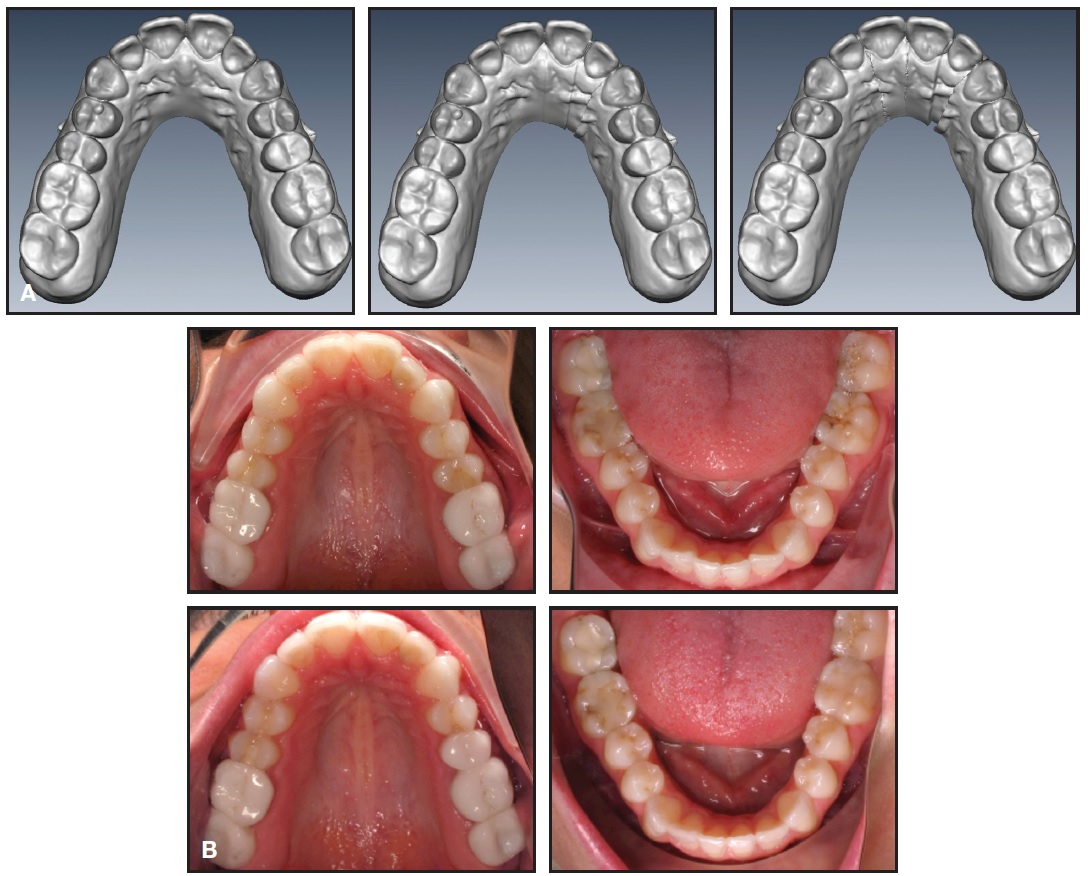
Fig. 9 A. Digital setups made with Orchestrate 3D for minor tooth movements. B. Clinical case treated with 3D-printed aligners fabricated in office.
Thermoformed aligners are easily made in the office using a material of the practitioner's choice. This cost-effective treatment is ideal for mild-to-moderate tooth movements, as compared to more expensive laboratory systems.
An acrylic appliance can be fabricated directly on a 3D-printed model after a separating medium has been applied; depending on the appliance, full palatal coverage may be needed. In contrast, an appliance that requires any soldering must be fabricated on stone models, because the heat of soldering (laboratory torches can reach temperatures as high as 3,000ºF) would deform or melt 3D-printed models--even those made from materials designed to withstand high temperatures. Therefore, the 3D-printed model must be duplicated in stone and made in the traditional manner. In the future, laboratories will likely have milling machines that will produce stone models directly from STL files through subtractive manufacturing.
Conclusion
Although 3D printing has yet to become commonplace in orthodontic practice, we expect it to follow a path similar to that of intraoral digital scanners. With the addition of a 3D printer, the orthodontist can achieve a completely digital workflow. Eliminating traditional impressions and stone models not only reduces clutter and storage requirements in the office, but enhances practice efficiency, improves appliance fit, allows model reuse, and results in more satisfied patients and staff members.
ACKNOWLEDGMENTS: The authors would like to thank Avi Cohen at Stratasys Ltd. and Al Siblani at EnvisionTEC for their assistance with this article. They are also grateful to Nancy McCullick in Dr. Groth's practice for producing the printer diagrams.
FOOTNOTES
- *Registered trademark of Align Technology, Inc., San Jose, CA; www.invisalign.com.
- **Trademark of ClearCorrect, Inc., Round Rock, TX; www.clearcorrect.com.
- ***3D Systems, Rock Hill, SC; www.3dsystems.com.
- †Stratasys Ltd., Minneapolis, MN; www.stratasys.com. PolyJet is a trademark. Objet Geometries is now a subsidiary of Stratasys.
- ‡EnvisionTEC, Dearborn, MI; www.envisiontec.com.
- ††Registered trademark of Texas Instruments Incorporated, Dallas, TX; www.ti.com.
- ‡‡Trademark of MakerBot, Brooklyn, NY; www.makerbot.com.
- §Trademark of Netfabb GmbH, Lupburg, Germany; www.netfabb.com.
- §§Registered trademark of Geomagic Solutions, Cary, NC; www.geomagic.com.
- §§§Orchestrate Orthodontic Technologies, Rialto, CA; www.orchestrate3d.com.
REFERENCES
- 1. Kravitz, N.D.; Groth, C.; Jones, P.E.; Graham, J.W.; and Redmond, W.R.: Intraoral digital scanners, J. Clin. Orthod. 48:337-347, 2014.
- 2. Shulman, H.; Spradling, D.; and Hoag, C.: Introduction to additive manufacturing, Ceram. Indus. 162:15-21, 2012.
- 3. Stoodley, M.A.; Abbott, J.R.; and Simpson, D.A.: Titanium cranioplasty using 3-D computer modelling of skull defects, J. Clin. Neurosci. 32:149-155, 1996.
- 4. Zein, I.; Hutmacher, D.W.; Tan, K.C.; and Teoh, S.H.: Fused deposition modeling of novel scaffold architectures for tissue engineering applications, Biomater. 23:1169-1185, 2002.
- 5. Van Noort, R.: The future of dental devices is digital, Dent. Mater. 28:3-12, 2012.
- 6. Jones, P.E.: 3D printing: What is it and how does it benefit tooth movement? J. Am. Acad. Cosmet. Orthod., Spring 2014.
- 7. Hull, C.: Apparatus for production of three-dimensional objects by stereolithography, U.S. Patent No. 4,575,330, March 11, 1986.
- 8. Kalpakjian, S. and Schmid, S.R.: Material-removal processes: Abrasive, chemical, electrical, and high-energy beams, in Manufacturing Engineering and Technology, 5th ed., Pearson Prentice Hall, Upper Saddle River, NJ, 2006, pp. 586-687.